Zero fossil fuels by 2050 without energy austerity
Richard Corin
Mark Diesendorf has mentioned (ERA Review v14 n4, p7) that “We will have to cut energy consumption 50-75% by 2050 while accelerating the renewable build. And that means lifestyle change driven by social policies.”
That is a big ask if “lifestyle change” means cooking, lighting, transport and thermal services such as “hot showers and cold beer” become expensive luxuries. Pushing people to do without energy services is likely to provoke a backslash that will undermine political will. Does this mean that civilisation is doomed? I had to take a closer look because such profound implications swing on an ambiguity about what the word “consumption” means. Does “lifestyle change” imply deprivation or is it merely a matter of electrification? Can energy consumption be slashed without cutting energy services?
The article is correct to say that technological change will not be able to halve the world’s CO2 emissions from fossil fuels by 2030. Logistics and lead times mean that 7 years is insufficient. Even with enthusiastic political will and unlimited investment, the vast majority of people needed to build the capacity to make the equipment and install the infrastructure to displace fossil fuel energy sources, are not even able to enrol in appropriate courses.
It has been left too late for humanity to replace 50% of fossil fuelled energy by 2030, so the only way that target can be met would be to effectively shut down civilisation or, to understate the problem somewhat, “we need to talk about cutting consumption”.
The longer goal of getting to zero by 2050 is viable, however, with a tradeoff between early efforts and future costs. And the best solution remains to replace fossil fuel energy with renewable electricity.
Replacing the world’s energy supply will require a whole lot of energy to implement. Consequently, any premature restriction of fossil fuel supplies will not only reduce real incomes via inflation, but also retard the transition to renewable energy at a time when everything that can be done should be done to accelerate it. It is likely that global emissions in 2030 will exceed those of 2020, in part because the world must build huge amounts of renewable energy equipment quickly if fossil fuels are to be eliminated by 2050.
Provided we have scaled up the industries needed for the twenty years after 2030, breaching the 2030 emissions target is no reason for despair. Replacing fossil fuels by 2050 remains feasible, provided the collective will exists, the natural resources are accessible and the EROI (Energy Return on Energy Invested) for reliable, balanced renewable energy systems is at least 8. Note that “energy invested” includes associated processes such as energy storage, mining, transmission lines and power electronics.
In a nutshell, the world’s capacity to make and install appropriate technology needs to be scaled up by 2030, around 6.5 times what it was in 2020. If it takes longer to ramp up production, the multiple has to be even larger in order to make up lost time. I’m not talking about demand side appliances such as EVs and heat pumps – but the renewable energy equipment industries. Multiplying the 2020 volume of manufacturing and installing renewable energy equipment by 6.5 times is about the right scale for 2030 – thereafter, growth of the industry can slow to match expected growth of electricity demand (around 1.9% pa ).
These estimates come from modelling a transition to renewable energy using the same International Energy Agency data as Mark Diesendorf’s Supplementary Material to his paper: Scenarios for mitigating CO2 emissions from energy supply in absence of CO2 removal [1] and on replacing every 100 joules of fossil fuelled combustion with only 36 joules of renewable electricity.
“Joules ain’t Joules, Sol”
The science of thermodynamics grew out of a desire to construct ever better steam engines, or “heat engines” in general. Combustion releases a lot of energy, but getting work out of heat is unavoidably lossy and inefficient.
Energy is not destroyed, but becomes increasingly dissipated and useless on its way to becoming ambient warmth. The surface heat of the Earth ultimately escapes the atmosphere and radiates out into space. Space remains very cold because the universe is expanding. Because energy is never lost, but degrades, it is rarely useful to sum the different types of energy without allowing for the quality of that energy in the context. This fact also helps to explain how we can be misled by aggregated energy data.
Heat is an inevitable waste product of all energy processes. Electrical energy is more suited to delivering efficient energy services than is combustion, because concentrated, controllable forms of energy deliver more useful work than employing a heat engine to exploit a temperature difference.
According to the IEA (the International Energy Agency) the average efficiency for the thermal generation of electricity is 36%, so 64% of the energy from burning fossil fuels is wasted. And we should not need to replace waste heat with precious electricity, so supplying renewable electricity reduces primary energy consumption by 64%. If electricity can replace thermal energy in the same ratio for other energy services, then “reducing consumption” need not mean slashing energy services at all!
In my modelling, I have assumed that every 36 joules of renewable electricity can replace 100 joules of fossil-fuelled heat. I have extended the replacement ratio for fossil-fuelled electricity to the whole system. This may turn out to be optimistic, but I believe it is reasonable.
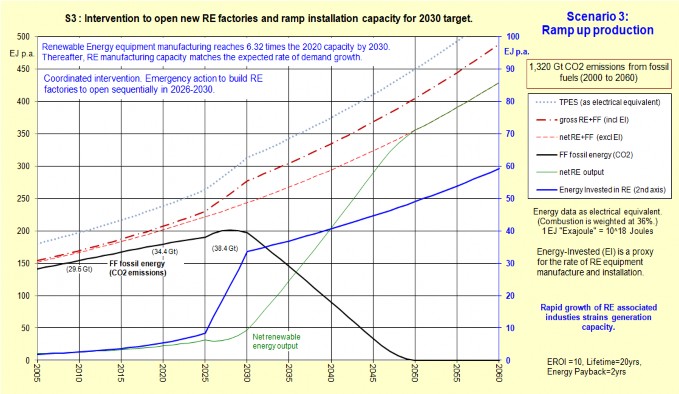
Internal Combustion Engines (ICE) in vehicles are twice as wasteful as thermal power stations, so electrifying transport has an even better replacement ratio. After accounting for losses in distribution and battery charging, each joule of electricity replaces over 4 joules of energy from burning petrol. Typical car efficiencies are: ICE 18%, HFC 30%, EV 76%.
Heat pump hot water systems concentrate thermal energy from the environment so that they typically deliver four joules of heat for each joule of electricity consumed. Doing the math reveals that a heat pump hot water service using renewable electricity uses only 9% of the primary energy consumed by a resistive heater powered from a thermal power station. This is how we can think about cutting consumption.
Although there are many examples where using appropriate electric technologies displaces more than 3 times the energy from burning fuels, there are also industrial processes in which the fuel itself acts as a chemical agent and in this case making a substitute fuel using electricity is not so efficient.
Examples include natural gas (mostly methane) which is conventionally used to make ammonia as a feedstock for nitrogenous fertilisers and other chemical products; coking coal is used to make steel; long haul aviation depends on the high energy density of kerosene (by mass and volume); while plastics are made from petroleum.
Replacing the fossil fuel feedstock requires a supply of biological hydrocarbons or green hydrogen – however, electrolysing water to produce hydrogen currently wastes around 30% of the electrical input energy. After that, there are distribution losses; energy is consumed compressing H2 gas into metal bottles, and the molecules are so small that they tend to leak through the walls of containers and pipes.
Volkswagen’s research on hydrogen-fuelled vehicles claims around half the source energy is lost before reaching the vehicle tank [2].
After wasting about half the energy of the original electricity, burning green hydrogen has the same thermodynamic losses as any other heat engine. But a hydrogen fuel cell is not a heat engine. By intervening in the chemistry of combustion, a HFC delivers 65% of the chemical energy in the form of electricity – however that is 65% of the remaining 50%, and the net result of 32.5% is not particularly good.
If there is going to be a reduction of per capita energy services, it will be manifested as price rises for nitrogenous fertiliser (i.e. food!), steel, air travel, cement and plastics – rather than electric heating and transport.
The ratio for replacing combustion with electricity matters, because it would be practically impossible to replace the hundreds of exajoules of fossil fuels burned on Earth every year on a joule for joule basis [note that one exajoule “EJ” is 1018 joules]. The substitution factor can also be expressed as an energy saving from electrification. If the substitution ratio happens to be 36%, then the energy saving is 64% (100%-36%). Many disagreements amongst friends will boil down to assumptions about efficiencies and the mix of energy technologies.
Quoting M.D.’s “Scenarios” paper: “Bogdanov et al. (2021) [3] calculate that the transition to 100% RE could provide 50% savings in TPES [Total Primary Energy Supply]”. This reference is an excellent paper, but I did not find a discussion of this 50% estimate.
In the Q&A section of ERA Review for Jul-Aug 2022, p10, M.D. suggests the replacement ratio of fossil energy to renewable electricity will be about two (a 50% saving) because much of the renewable electricity will go into green hydrogen. A paper by Jacobson et al (2018) [4] puts the energy saving at about 58%, and it’s reasonable to assume that the quest for energy efficiency will reduce this level of waste further. For example, if electric container ships are like EVs, then energy costs will be 2.5 times higher for hydrogen fuel cells than for batteries.
The 50-64% primary energy saving we receive from electrification and renewable energy provides most of the “5075%” energy consumption cuts prescribed in the ERAR article, so it does not seem to be a problem that technology cannot solve (i.e. requiring severe and inevitable lifestyle change). Confusion over what constitutes energy savings can lead us to an unduly pessimistic prognosis.
Climate change may well turn out to be worse than expected, but it remains feasible to end burning fossil fuels by 2050 without sacrificing energy services.
What really matters is the total amount of CO2 accumulated in the biosphere. The scenarios I have modelled suggest that 21st century emissions will exceed 1250Gt of CO2 from burning fossil fuels.
As 2023 begins, only seven years are available for ramping up the global renewable energy equipment capacity to around 6.5 times its 2020 magnitude.
I strongly recommend industrial intervention, as modelled in “scenario 3” [illustrated], which shows what may be possible, but reveals a problematic bump in CO2 emissions, ironically, due to the rapid growth of the renewable energy sector. To avoid any need to build additional fossil fuel infrastructure, “scenario 4”, employs a 12-13% cut in retail energy demand to make energy available for boosting the RE sector.
When fossil fuels have been replaced and it becomes illegal to burn them, the scale of real economic activity will depend on the supply of renewable energy and the efficiency of our technology. We will need additional energy to up-cycle wastes into raw materials and, probably, to remove CO2 from the atmosphere.
There is an historical linear relationship between primary energy consumption and GDP. When the major energy source is no longer fossil fuels, but renewable electricity, this ratio will be change significantly, reflecting a 5064% reduction of energy per dollar of GDP.
A text document containing hyperlinks to useful references and an interactive excel spreadsheet featuring seven hypothetical scenarios is available at the ERA website.
References
- https://www.tandfonline.com/eprint/D8ZAEUFKUDFTKI94QQJ/full
- https://www.volkswagenag.com/en/news/stories/2019/08/hydrogen-or-battery–that-is-the-question.html
- https://www.sciencedirect.com/science/article/pii/S0360544221007167
- https://web.stanford.edu/group/efmh/jacobson/Articles/I/CombiningRenew/WorldGridIntegration.pdf